Let's
learn, What is current transformer? and how
does the current transformer works? with different types of CT
connections.

POLE TYPE CURRENT TRANSFORMER
what is current transformer?
The term current transformer is used to describe a piece of equipment consisting of pairs of mutually coupled windings mounted around a core, usually of magnetic materials. such transformers are normally used to step down high current flowing in their primary windings to lower levels suitable for feeding to measuring and protective equipment.
Another good definition of what is a current transformer? by
"James H Harlow"
The current transformer
is often treated as a "BlackBox". It is a transformer that is
governed by the laws of electromagnetic induction:
ε
=k β Ac Nf (7.12)
ε = induced voltage
k =
constant of proportionality
β = flux density
Ac =
cross-sectional area
N =
Turns
F = frequency
How does the current transformer works?
As previously stated, the CT is employed in series with the circuit to be monitored and it's this difference that results in its ambiguous description. the primary winding is to bring a constant current source of supply through a low impedance loop. Due to this low impedance, current passes through it with very little regulation. The CT operates on the ampere-turn principle:
primary ampere-turns = secondary ampere-turns, or
(7.13)
Since there is energy loss during transformation, this loss can be explained in
ampere-turns: primary ampere-turns, magnetizing ampere- turns= secondary
ampere-turns, or

(7.14)
The CT is not
voltage-dependent but it is voltage limited. As current passes through and
impedance, a voltage is developed, V=IZ. As this occurs, energy is
depleted from the primary supply, thus acting as a shunt. This
depletion of energy results in CT errors.
As the secondary impedance
increases, the voltage proportionally increases. Thus the limit of the CT
is magnetic saturation, a condition where the CT core flux can no longer
support the increased voltage demand.
At this point, nearly all of the
available energy is going into the CT core, leaving none to support the
secondary circuit.
Let's learn how does the current transformer work with the help of the equivalent circuit.
To understand how does the current transformer works, one has to first understand the basic equivalent circuits. These transformers behave similarly to all two winding transformers and may be represented by the well-known circuit shown in figure 2.3(a) which is based on the winding directions and current and voltage polarities shown in figure 2.3(b).
Because the connected CT burdens are normally of low impedance, the secondary output VA and voltage are relatively low, typically of a maximum of 20VA and 20V for a current transformer with a secondary winding rated at 1 ampere.
The voltage across the primary winding, because of the turns ratio, is not therefore likely to exceed a fraction of 1 volt, a negligible value relative to the rated voltage of the primary circuit.
For this reason, the equivalent circuit of a current transformer can be simplified to that shown in figure 2.4. This circuit can be made to represent any current transformer operating with any CT burden and primary current under either steady-state or transient conditions.

Fig 2.3 Equivalent circuit of a current transformer

Fig 2.4 Equivalent circuit of a current transformer referred to the secondary
It will be seen that the exciting current Ie is dependent on the exciting impedance, presented by Rl in parallel with lm and the secondary E.M.F (Es) is needed to drive the secondary current Is through the total secondary circuit impedance.
Because the secondary current of a current transformer may vary over a wide range, that is from zero under no-load conditions to very large values but when there is a fault on the primary circuit the secondary E.M.F and excitation current may also vary greatly and in this respect, the behavior is very different from that of voltage transformers.
Because of the non-linearity of the excitation characteristics of the magnetic materials used for current transformer (CT)cores, the exciting impedance of a given current transformer is not constant, both the magnetizing inductance Lm and loss resistance R1 varying with the CT core flux needed to provide the secondary E.M.F es.
Allowance may be made for this non-linearity, if necessary, when determining the behavior of a particular Transformer under specified conditions, calculation than being done using step-by-step or other methods. If great accuracy is not required, however, simplifications can be affected by assigning constant values, the averages over a cycle, to Rl and Lm.
An alternative method of representing a current transformer is to employ the concept of mutual inductance Mps. By definition and based on the conventions used above:
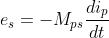
when there is zero current in the secondary winding. It has been shown that the circuit shown in figure 2.5 is equally as satisfactory a model as that shown in figure 2.4.
The self-inductance, Lss of a secondary winding is given by:
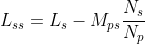
in which Ls is the leakage inductance used in the circuit of figure 2.4. It is clear therefore that the value of Ls for a given Transformer may be determined experimentally by measuring itself Lss and mutual inductances Mps.
Saturation curve in Current transformer
The saturation curve,
often called the secondary excitation curve, is a plot of secondary exciting
voltage versus secondary exciting current drawn on log-log paper. The units are
in RMS with the understanding that the applied voltage is sinusoidal.
This characteristic
defines the CT core properties after the stress relief annealing process. It can
be demonstrated by a test that CT cores processed in the same manner will always
follow this characteristic within the specified tolerances. Figure 7.14 shows a
typical characteristic of a 600:5 multi ratio CT. The knee point is indicated
by the dashed line. Since the voltage is proportional to the turns,
the volts per turn at the knee are constant.
The tolerances are 95% of
saturation voltage for any exciting current above the knee point and 125%
of exciting current for any voltage below the knee point.
These
tolerances, however, can create a discontinuity about the knee of the
curve, which is illustrated in figure 7.14. since the tolerance is referenced
at the knee point, It is possible to have a characteristic that is shifted to
the right of the nominal, within tolerance below the knee point.
But
careful inspection shows that a portion of the characteristic will exceed the
tolerance above the knee point. for this reason manufacturers, typical curves
may be somewhat conservative to avoid this situation in regards to field
testing. some manufacturers will provide actual test data that may provide the
relay Engineer with more useful information.
knowing the secondary winding
resistance and the excitation characteristic, the user can calculate the
expected RCF under various conditions. using this type of curve is only valid
for non-metering applications. the required voltage needed from the CT must be
calculated using the total circuit impedance and the anticipated secondary
current level. The corresponding exciting current is read from the curve and
used to approximate the anticipated errors.
where,
Vex
= secondary excitation voltage required at fault level
Isf
= secondary fault current
Iex
= secondary exciting current at Vex, obtained from curve
ZT
= is the total circuit impedence
Rs
= secondary winding resistance
RB = secondary burden
resistance
XB = secondary burden
reactance
RCF
= ratio correction factor
RE
= ratio error
In the world of protection, the best
situation is to avoid saturation entirely. this can be achieved by sizing the
CT knee point voltage to be greater than Vex, but this may not be the
most practical approach. This could force the CT physical size to substantially
increase as well as cause dielectric issues. There must be some reasonable
trade-off to reach a desirable condition. equation 7.15 provides the voltage
necessary to avoid AC saturation. if there is an offset that will introduce a
DC component, then the system X/R ratio must be factored in:
The saturation
factor Ks is the ratio of the knee point voltage to the required secondary
voltage Vex. it is an index of how close to saturation a CT will be in a given
application. Ks is used to calculate the time, a CT will saturate under certain
conditions:
Where,
Ts = Time to saturate
ω = 2πf, where f is the system frequency
Ks = saturation factor,
R = primary system resistance at
the point of fault
X = primary system reactance at
point of fault
Ln = natural log function
CT thermal rating factor in current transformer
The continuous
current rating factor is given at a reference ambient temperature,
usually 30-degree Celcius. The standard convention is that the average
temperature rise will not exceed 55 degree Celsius for general purpose use, but
it can be any rise shown in table 7.8. from this CT rating factor, a given CT can
be derated for use in higher ambient temperature from the following
relationship:

(7.21)
which can be simplified and written as:

(7.22)
where,
RFnew = Desired rating factor at
some other ambient temperature,
RF std = Reference rating factor at
30 degree Celcius.
AMB new = desired ambient temperature at < 85 degree
celcius.
Equation 7.22 is
valid only for 55 degrees Celsius rise ratings and maximum ambient less than 85
degrees Celsius.
The rating factor
ensures that the CT will not exceed its insulation class rating. this
expression follows the loading curves of figure 1 in IEEE C57.13.It assumes
that the average winding temperature rise is proportional to the current
squared and those CT core watt losses are insignificant under continuous operating
conditions.
Conversely,
this expression will work for ambient temperature lower than 30 degrees
Celsius, but in this situation, the rating factor will be higher. the
concern is not with exceeding the insulation system but, rather with the chance
of increasing the errors of the CT accuracy limits. A number of factors can
affect this, such as CT core material and compensation methods used. it is
best to consult the manufacturer for overrating performances.
Another
consideration is the stated rating factor versus the actual temperature rise.
in most cases, the stated rating factor may be quite less than the
permitted 55 degrees Celsius rise allowance. for example, the actual
rating factor may be 2.67, but the stated rating factor is 2.0. if the
actual rise data were known, then equation 7.22 could be rewritten as:

(7.23)

Figure 7.15 CT derating chart
RFactual
= rating factor at an actual temperature
∆Trise = actual measured temperature
rise.
This may be more useful when operating a
CT at a Higher ambient, where there is a need for the maximum rating factor. For example, if the CT has an actual rating of 2.67 based on temperature rise
data but is only rated 2.0 and if it is desired to use the CT at 50
degree Celsius ambient with the stated rating factor 2.0, then this unit
should work within its insulation rating and within its stated accuracy class.
If a higher temperature class insulation system is provided, then the
rise must be in compliance with that class per table 7.8. In some cases, the
temperature class is selected for the environment rather than the actual
temperature rise of the CT.
Open circuit conditions in current transformer
The CT functions best with the minimum CT
burden possible, which would be its internal impedance.
This can only be accomplished by applying a short circuit across
the secondary terminals. since the CT core MMF acts like a
shunt, with no load connected to it's secondary, the MMF becomes the primary
current, thus dividing the CT into hard saturation. with no load on the
secondary to control the voltage, the winding develops an
extremely high peak voltage.
This voltage
can be in the thousands or even tens of thousands of volts. This situation puts the winding under incredible stress, ultimately leading
to failure. This could result in damage to other equipment or present
a hazard to personnel.it is for this reason that the secondary
circuit should never be open. it must always have a load connected.
If it is installed to the primary but not in use, then the terminals should be
shorted until it is to be used. most manufacturers ship CTs with a shorting strap
for wire across the secondary terminals. the CT winding must be able
to withstand 3500 Vpeak for 1 minute under open-circuit conditions. if the
voltage can exceed this level, then it is recommended that overvoltage
protection be used.
Overvoltage protection in current
transformer
Under load,
the CT voltage is limited. The level of this voltage depends on the turns and CT
core cross-sectional area. the user must evaluate the limits of the CT
burdens connected to ensure equipment safety. sometimes protective devices
are used on the secondary side to maintain safe levels of voltage. these
devices are also incorporated to protect the CT during an open circuit
condition.
In metering
applications, it is possible for such a device to introduce a direct current
across the winding that could saturate the CT core or leave
it in some state of residual flux. in High Voltage equipment, arrestors maybe
used to protect the primary winding from high voltage spikes produced
by switching transients or lighting.
Residual magnetism in current transformer
Residual magnetism, residual
flux, or remanence refers to the amount of stored, or trapped, flux
in the CT core. this can be introduced during heavy saturation or
with the presence of some DC components.
figure 7.16 shows a typical B-H curve for Silicon iron driven
into hard saturation.
The point at which the curve
crosses zero force, identified by +Bres, represents residual
flux. If at some point the CT is disconnected from the source, this flux
will remain in the magnetic CT core until another source becomes
present. If a fault current drove the CT into saturation, when the supply
current resumes normal levels, the CT core would contain some
residual components.
Residual flux does not
gradually decay but remains constant once steady-state equilibrium has been
reached. Under normal conditions, the minor B-H loop must be high enough
to remove the residual component. if it is not, then it will remain present
until another fault occurrence takes place. the effective result could be a
reduction of the saturation flux.

Fig 7.16 Typical B-H curve for Si - Fe steel
However, if a transient of opposite Polarity
occurs, saturation is reduced with the assistance of the residual.
conversely, the magnitude of the residual is also based on the polarity of
the transient and the phase relationship of the flux and current. whatever the
outcome, the result would cause a delayed response to the connected
relay.
It has been observed
that in a tape-owned toroidal CT core as much as 85% of saturation flux could be
left in the CT core as a residual component. the best way to remove residual flux
is to demagnetize the CT core.
This is not always
practical. the user could select a CT with a class that is twice that required.
this may not eliminate residual flux, but it will certainly reduce the
magnitude. the use of hot-rolled Steel may inherently reduce the residual
component to 40 to 50% of saturation flux.
Another way of reducing residual
magnetism is to use and Air gapped CT core. normally the introduction of a gap
that is, say 0.01% of CT core circumference could limit the residual flux to
about 10% of the saturation flux. referring to figures 7.16, a typical
B-H loop for and Air gapped CT core is shown.
The drawback is significantly higher
exciting current and lower saturation levels, as can be seen in figure
7.3. to overcome the high exciting current, the CT core would be made
larger. that coupled with the gap would increase its overall cost. This type
of CT core construction is often referred to as a linearized CT core.
Let's understand current transformer( CT)
connections
As previously mentioned, some devices are sensitive to the
direction of current flow. It is often critical in three-phase schemes to
maintain proper phase shifting. Residually CT connections in a three-phase ground fault scheme sum to
zero when the phases are balanced.
The
reversed polarity of a CT could cause a ground fault relay to trip under a
normal balance condition. Another scheme to detect zero-sequence faults use one
CT to simultaneously monitor all leads and neutral in differential protection
schemes. current source phase and magnitude are compared.
The reverse polarity of a CT could
effectively double the phase current flowing into the relay, thus causing a
nuisance tripping of a relay. when two CTs are driving a three-phase ammeter
through a switch, a reversed CT could show 1.73 times the monitored current
flowing in the unmonitored circuit.
In CT
connections, the wye-connected secondary circuit is the most commonly
used. the CT will reproduce positive negative and zero sequence elements as
they occur in the primary circuit. in the delta-connected secondary, the
zero sequence components are filtered and left to circulate in the delta.

Figure 7.17 (a) over current and ground fault protection scheme (b)differential protection scheme (c)zero-sequence scheme
This is a common scheme for differential
protection of delta-wye transformers. A general rule of thumb is to construct the current transformer (CT) connections for secondaries in wye when they are on the Delta winding of a transformer
and, conversely, connect the secondaries in Delta when they are on
the wye winding of the transformer.
Table of contents
Transformer - Working principle
Transformer-Types
Transformer-Elementary theory of an idle transformer, Emf Equation, voltage transformation ratio, transformer on no load
Transformer-ON Load
Transformer-Transformer with winding resistance but no Leakage reactance
Transformer-Equivalent resistance and MagneticLeakage
Transformer- resistance and Leakage Reactance
Transformer-Simplified diagram
Transformer-Total approximate voltage drop in a transformer,Exact voltage drop and its equivalent circuit
Transformer-Open-circuit test(no load test) and short circuit test(impedence test),seperation of core losses
Transformer-Why transformer rating is in KVA?
Transformer-Regulation
Transformer-percentage,resistance,reactance,impedence
Transformer-Kapp regulation diagram
Transformer-Sumpner back to back test
Transformer-Losses in a transformer
Transformer-Efficiency and condition for max efficiency
Transformer-All day Efficiency
Transformer-Auto Transformer
Transformer-Conversion of 2-windinding transformer into Auto-Transformer
Transformer-Parallel operation of single-phase Transformers
Transformer-Three Phase Transformer
Transformer-Star-Star Connection
Transformer-Delta-Delta Connection
Transformer- Y-DELTA connection, DELTA-Y connection, OPEN - DELTA Connection
Transformer-Scott connection or T-T connection
Transformer-Three phase to Two phase conversion
Transformer-Parallel Operation of Three phase Transformers
Questions on current transformer
1.what is the purpose of a current transformer?
Current transformers are made to measure, or monitor, the flow of current within the ac power circuit. They're very useful in high power circuits, where the flow of current is large, i.e more current than the ratings of so-called self-contained current meters. Other applications relate to overcurrent and undercurrent relaying for power circuit protection, such as, within the power lead of an inverter or converter.
2.what do you mean by current transformer?
A current transformer is an electrical machine, that works to measure high AC which is not supposed to be sensed or passed through normal ammeters and current coils of wattmeter, energy meters, etc.
A current transformer basically includes a primary coil of single or more turns of heavy cross-sectional area. In some, the bar carrying a high current can act as a primary. This is joined in series with the circuit carrying a high current. The secondary of the AC transformer is constructed up from a large number of turns of fine wire having a small cross-sectional area. This is usually rated for Five ampere. This is coupled with the coil of the normal range ammeter.
4. What are the different types of current Transformers?
There are 5 types of current Transformers
1.Portable type
2.Outdoor type
3.Indoor type
4.Bushing type
5.Multi-ratio type
Indoor type current transformers are of two types1.Wound type
2.Bar and window type
Many outdoor type current transformers are actually used for higher voltage circuits. In the same way, Indoor types of current transformers are employed for low voltage circuits.
In a wound core type, the primary coil consisting of several turns is wound over the core. The core is mostly made up of a Ni-Fe alloy or specially treated steel and is used in such a way that the flux flowing always in the direction of grains as produced by Rolling the sheet metal. This improves the magnetic properties and helps in decreasing the ratio and phase angle errors.
Current Transformers using hot rolled Steel are made up of stacks of ring stampings which are insulated by means of the end-collars and circumferential wrap of pressboard protect the secondary winding from mechanical damage and also act as an insulating medium.
In a bar-type current transformer, the primary coil consists of a bar of suitable size that forms an integral part of the transformer as shown in figure 5.6. In bar type, the primary ampere-turns are numerically the same as the primary current. However, at a low current rating, the accuracy decreases because the magnetization of law demands a larger fraction of the total ampere-turns.
In the case of wound core type construction, higher accuracy can be achieved because of the higher value of primary ampere-turns.
The bushing type transformer is similar to the bar type. The core and secondary are mounted around a single primary conductor.
The secondary coil is placed over a circular core which is installed in the high voltage busing of a circuit breaker or power transformer.
The primary coil is the conductor in the bushing. In Precision type portable current Transformers, the choice of ratios can be obtained by changing the links on the top plate.
In laboratory-type current Transformers, the variable-ratio can be obtained by using the following methods:
1. The variable-ratio can be obtained by using the series-parallel connection of the primary coil. It should be ensured that the various coil sections used must have the same characteristics.
2. It can be obtained by using tappings on the primary or the secondary coil. This method provides a higher degree of flexibility. but when applied to the primary side, much of the copper space is left unused except on the lowest range. when used on the secondary-coil side, working ampere-turns are changed.

Fig 5.6 CURRENT TRANSFORMER WITH BAR TYPE PRIMARY
3.Using a variable primary formed by threading any desired number of turns of cable through the core. For this purpose, a ring core and a toroidal Core secondary is generally used.
Split core current TransformersIn some applications, it is desirable that the core of the current transformer is split into two halves. The conductor which is already fixed in location may be encircled thus making a bar primary type of current transformer.
It is also called a clamp-type ammeter in which the instrument and split-core transformer are combined to form a single unit. They have a self-aligning Hing Limb of split-core which is operated by a spring-loaded trigger to spread the core into two halves and clip around the current-carrying conductor as shown in figure 5.7.

Fig 5.7 SPLIT-CORE CURRENT TRANSFORMER
This arrangement avoids the necessity of breaking the circuit for the purpose of measurement. For increasing the range shunts are used and by varying the shunt-resistance of the milli-ammeter circuit, current ranges from 0 to 5 ampere to 0 to 600 ampere can be obtained.
Let's learn, What is current transformer? and how does the current transformer works? with different types of CT connections.
![]() |
POLE TYPE CURRENT TRANSFORMER |
what is current transformer?
The term current transformer is used to describe a piece of equipment consisting of pairs of mutually coupled windings mounted around a core, usually of magnetic materials. such transformers are normally used to step down high current flowing in their primary windings to lower levels suitable for feeding to measuring and protective equipment.Another good definition of what is a current transformer? by "James H Harlow"
The current transformer is often treated as a "BlackBox". It is a transformer that is governed by the laws of electromagnetic induction:
ε =k β Ac Nf (7.12)
ε = induced voltage
k = constant of proportionality
β = flux density
Ac = cross-sectional area
N = Turns
F = frequency
How does the current transformer works?
As previously stated, the CT is employed in series with the circuit to be monitored and it's this difference that results in its ambiguous description. the primary winding is to bring a constant current source of supply through a low impedance loop. Due to this low impedance, current passes through it with very little regulation. The CT operates on the ampere-turn principle:
primary ampere-turns = secondary ampere-turns, or
(7.13)
Since there is energy loss during transformation, this loss can be explained in
ampere-turns: primary ampere-turns, magnetizing ampere- turns= secondary
ampere-turns, or
(7.14)
The CT is not
voltage-dependent but it is voltage limited. As current passes through and
impedance, a voltage is developed, V=IZ. As this occurs, energy is
depleted from the primary supply, thus acting as a shunt. This
depletion of energy results in CT errors.
As the secondary impedance
increases, the voltage proportionally increases. Thus the limit of the CT
is magnetic saturation, a condition where the CT core flux can no longer
support the increased voltage demand.
At this point, nearly all of the
available energy is going into the CT core, leaving none to support the
secondary circuit.
Let's learn how does the current transformer work with the help of the equivalent circuit.
To understand how does the current transformer works, one has to first understand the basic equivalent circuits. These transformers behave similarly to all two winding transformers and may be represented by the well-known circuit shown in figure 2.3(a) which is based on the winding directions and current and voltage polarities shown in figure 2.3(b).
Because the connected CT burdens are normally of low impedance, the secondary output VA and voltage are relatively low, typically of a maximum of 20VA and 20V for a current transformer with a secondary winding rated at 1 ampere.
The voltage across the primary winding, because of the turns ratio, is not therefore likely to exceed a fraction of 1 volt, a negligible value relative to the rated voltage of the primary circuit.
For this reason, the equivalent circuit of a current transformer can be simplified to that shown in figure 2.4. This circuit can be made to represent any current transformer operating with any CT burden and primary current under either steady-state or transient conditions.
![]() |
Fig 2.3 Equivalent circuit of a current transformer |
![]() |
Fig 2.4 Equivalent circuit of a current transformer referred to the secondary |
Because the secondary current of a current transformer may vary over a wide range, that is from zero under no-load conditions to very large values but when there is a fault on the primary circuit the secondary E.M.F and excitation current may also vary greatly and in this respect, the behavior is very different from that of voltage transformers.
Because of the non-linearity of the excitation characteristics of the magnetic materials used for current transformer (CT)cores, the exciting impedance of a given current transformer is not constant, both the magnetizing inductance Lm and loss resistance R1 varying with the CT core flux needed to provide the secondary E.M.F es.
Allowance may be made for this non-linearity, if necessary, when determining the behavior of a particular Transformer under specified conditions, calculation than being done using step-by-step or other methods. If great accuracy is not required, however, simplifications can be affected by assigning constant values, the averages over a cycle, to Rl and Lm.
An alternative method of representing a current transformer is to employ the concept of mutual inductance Mps. By definition and based on the conventions used above:
when there is zero current in the secondary winding. It has been shown that the circuit shown in figure 2.5 is equally as satisfactory a model as that shown in figure 2.4.
The self-inductance, Lss of a secondary winding is given by:
in which Ls is the leakage inductance used in the circuit of figure 2.4. It is clear therefore that the value of Ls for a given Transformer may be determined experimentally by measuring itself Lss and mutual inductances Mps.
Saturation curve in Current transformer
The saturation curve, often called the secondary excitation curve, is a plot of secondary exciting voltage versus secondary exciting current drawn on log-log paper. The units are in RMS with the understanding that the applied voltage is sinusoidal.
This characteristic defines the CT core properties after the stress relief annealing process. It can be demonstrated by a test that CT cores processed in the same manner will always follow this characteristic within the specified tolerances. Figure 7.14 shows a typical characteristic of a 600:5 multi ratio CT. The knee point is indicated by the dashed line. Since the voltage is proportional to the turns, the volts per turn at the knee are constant.
The tolerances are 95% of saturation voltage for any exciting current above the knee point and 125% of exciting current for any voltage below the knee point.
These tolerances, however, can create a discontinuity about the knee of the curve, which is illustrated in figure 7.14. since the tolerance is referenced at the knee point, It is possible to have a characteristic that is shifted to the right of the nominal, within tolerance below the knee point.
But careful inspection shows that a portion of the characteristic will exceed the tolerance above the knee point. for this reason manufacturers, typical curves may be somewhat conservative to avoid this situation in regards to field testing. some manufacturers will provide actual test data that may provide the relay Engineer with more useful information.
knowing the secondary winding resistance and the excitation characteristic, the user can calculate the expected RCF under various conditions. using this type of curve is only valid for non-metering applications. the required voltage needed from the CT must be calculated using the total circuit impedance and the anticipated secondary current level. The corresponding exciting current is read from the curve and used to approximate the anticipated errors.
where,
Vex = secondary excitation voltage required at fault level
Isf = secondary fault current
Iex = secondary exciting current at Vex, obtained from curve
ZT = is the total circuit impedence
Rs = secondary winding resistance
RB = secondary burden resistance
XB = secondary burden reactance
RCF = ratio correction factor
RE = ratio error
In the world of protection, the best situation is to avoid saturation entirely. this can be achieved by sizing the CT knee point voltage to be greater than Vex, but this may not be the most practical approach. This could force the CT physical size to substantially increase as well as cause dielectric issues. There must be some reasonable trade-off to reach a desirable condition. equation 7.15 provides the voltage necessary to avoid AC saturation. if there is an offset that will introduce a DC component, then the system X/R ratio must be factored in:
The saturation factor Ks is the ratio of the knee point voltage to the required secondary voltage Vex. it is an index of how close to saturation a CT will be in a given application. Ks is used to calculate the time, a CT will saturate under certain conditions:
Where,
Ts = Time to saturate
ω = 2πf, where f is the system frequency
Ks = saturation factor,
R = primary system resistance at the point of fault
X = primary system reactance at point of fault
Ln = natural log function
CT thermal rating factor in current transformer
The continuous current rating factor is given at a reference ambient temperature, usually 30-degree Celcius. The standard convention is that the average temperature rise will not exceed 55 degree Celsius for general purpose use, but it can be any rise shown in table 7.8. from this CT rating factor, a given CT can be derated for use in higher ambient temperature from the following relationship:
(7.21)
which can be simplified and written as:
(7.22)
where,
RFnew = Desired rating factor at some other ambient temperature,
RF std = Reference rating factor at 30 degree Celcius.
AMB new = desired ambient temperature at < 85 degree celcius.
Equation 7.22 is valid only for 55 degrees Celsius rise ratings and maximum ambient less than 85 degrees Celsius.
The rating factor ensures that the CT will not exceed its insulation class rating. this expression follows the loading curves of figure 1 in IEEE C57.13.It assumes that the average winding temperature rise is proportional to the current squared and those CT core watt losses are insignificant under continuous operating conditions.
Conversely, this expression will work for ambient temperature lower than 30 degrees Celsius, but in this situation, the rating factor will be higher. the concern is not with exceeding the insulation system but, rather with the chance of increasing the errors of the CT accuracy limits. A number of factors can affect this, such as CT core material and compensation methods used. it is best to consult the manufacturer for overrating performances.
Another consideration is the stated rating factor versus the actual temperature rise. in most cases, the stated rating factor may be quite less than the permitted 55 degrees Celsius rise allowance. for example, the actual rating factor may be 2.67, but the stated rating factor is 2.0. if the actual rise data were known, then equation 7.22 could be rewritten as:
(7.23)
![]() |
Figure 7.15 CT derating chart |
RFactual = rating factor at an actual temperature
∆Trise = actual measured temperature rise.
This may be more useful when operating a CT at a Higher ambient, where there is a need for the maximum rating factor. For example, if the CT has an actual rating of 2.67 based on temperature rise data but is only rated 2.0 and if it is desired to use the CT at 50 degree Celsius ambient with the stated rating factor 2.0, then this unit should work within its insulation rating and within its stated accuracy class.
If a higher temperature class insulation system is provided, then the rise must be in compliance with that class per table 7.8. In some cases, the temperature class is selected for the environment rather than the actual temperature rise of the CT.
Open circuit conditions in current transformer
This voltage
can be in the thousands or even tens of thousands of volts. This situation puts the winding under incredible stress, ultimately leading
to failure. This could result in damage to other equipment or present
a hazard to personnel.it is for this reason that the secondary
circuit should never be open. it must always have a load connected.
If it is installed to the primary but not in use, then the terminals should be
shorted until it is to be used. most manufacturers ship CTs with a shorting strap
for wire across the secondary terminals. the CT winding must be able
to withstand 3500 Vpeak for 1 minute under open-circuit conditions. if the
voltage can exceed this level, then it is recommended that overvoltage
protection be used.
Overvoltage protection in current transformer
Under load,
the CT voltage is limited. The level of this voltage depends on the turns and CT
core cross-sectional area. the user must evaluate the limits of the CT
burdens connected to ensure equipment safety. sometimes protective devices
are used on the secondary side to maintain safe levels of voltage. these
devices are also incorporated to protect the CT during an open circuit
condition.
In metering
applications, it is possible for such a device to introduce a direct current
across the winding that could saturate the CT core or leave
it in some state of residual flux. in High Voltage equipment, arrestors maybe
used to protect the primary winding from high voltage spikes produced
by switching transients or lighting.
Residual magnetism in current transformer
Residual magnetism, residual
flux, or remanence refers to the amount of stored, or trapped, flux
in the CT core. this can be introduced during heavy saturation or
with the presence of some DC components.
figure 7.16 shows a typical B-H curve for Silicon iron driven
into hard saturation.
The point at which the curve
crosses zero force, identified by +Bres, represents residual
flux. If at some point the CT is disconnected from the source, this flux
will remain in the magnetic CT core until another source becomes
present. If a fault current drove the CT into saturation, when the supply
current resumes normal levels, the CT core would contain some
residual components.
Residual flux does not gradually decay but remains constant once steady-state equilibrium has been reached. Under normal conditions, the minor B-H loop must be high enough to remove the residual component. if it is not, then it will remain present until another fault occurrence takes place. the effective result could be a reduction of the saturation flux.
![]() |
Fig 7.16 Typical B-H curve for Si - Fe steel |
It has been observed that in a tape-owned toroidal CT core as much as 85% of saturation flux could be left in the CT core as a residual component. the best way to remove residual flux is to demagnetize the CT core.
This is not always practical. the user could select a CT with a class that is twice that required. this may not eliminate residual flux, but it will certainly reduce the magnitude. the use of hot-rolled Steel may inherently reduce the residual component to 40 to 50% of saturation flux.
Another way of reducing residual magnetism is to use and Air gapped CT core. normally the introduction of a gap that is, say 0.01% of CT core circumference could limit the residual flux to about 10% of the saturation flux. referring to figures 7.16, a typical B-H loop for and Air gapped CT core is shown.
The drawback is significantly higher exciting current and lower saturation levels, as can be seen in figure 7.3. to overcome the high exciting current, the CT core would be made larger. that coupled with the gap would increase its overall cost. This type of CT core construction is often referred to as a linearized CT core.
Let's understand current transformer( CT) connections
As previously mentioned, some devices are sensitive to the
direction of current flow. It is often critical in three-phase schemes to
maintain proper phase shifting. Residually CT connections in a three-phase ground fault scheme sum to
zero when the phases are balanced.
The
reversed polarity of a CT could cause a ground fault relay to trip under a
normal balance condition. Another scheme to detect zero-sequence faults use one
CT to simultaneously monitor all leads and neutral in differential protection
schemes. current source phase and magnitude are compared.
The reverse polarity of a CT could
effectively double the phase current flowing into the relay, thus causing a
nuisance tripping of a relay. when two CTs are driving a three-phase ammeter
through a switch, a reversed CT could show 1.73 times the monitored current
flowing in the unmonitored circuit.
In CT
connections, the wye-connected secondary circuit is the most commonly
used. the CT will reproduce positive negative and zero sequence elements as
they occur in the primary circuit. in the delta-connected secondary, the
zero sequence components are filtered and left to circulate in the delta.
![]() |
Figure 7.17 (a) over current and ground fault protection scheme (b)differential protection scheme (c)zero-sequence scheme
|
This is a common scheme for differential protection of delta-wye transformers. A general rule of thumb is to construct the current transformer (CT) connections for secondaries in wye when they are on the Delta winding of a transformer and, conversely, connect the secondaries in Delta when they are on the wye winding of the transformer.
Table of contents
Transformer - Working principle
Transformer-Types
Transformer-Elementary theory of an idle transformer, Emf Equation, voltage transformation ratio, transformer on no load
Transformer-ON Load
Transformer-Transformer with winding resistance but no Leakage reactance
Transformer-Equivalent resistance and MagneticLeakage
Transformer- resistance and Leakage Reactance
Transformer-Simplified diagram
Transformer-Total approximate voltage drop in a transformer,Exact voltage drop and its equivalent circuit
Transformer-Open-circuit test(no load test) and short circuit test(impedence test),seperation of core losses
Transformer-Why transformer rating is in KVA?
Transformer-Regulation
Transformer-percentage,resistance,reactance,impedence
Transformer-Kapp regulation diagram
Transformer-Sumpner back to back test
Transformer-Losses in a transformer
Transformer-Efficiency and condition for max efficiency
Transformer-All day Efficiency
Transformer-Auto Transformer
Transformer-Conversion of 2-windinding transformer into Auto-Transformer
Transformer-Parallel operation of single-phase Transformers
Transformer-Three Phase Transformer
Transformer-Star-Star Connection
Transformer-Delta-Delta Connection
Transformer- Y-DELTA connection, DELTA-Y connection, OPEN - DELTA Connection
Transformer-Scott connection or T-T connection
Transformer-Three phase to Two phase conversion
Transformer-Parallel Operation of Three phase Transformers
Questions on current transformer
1.what is the purpose of a current transformer?
Current transformers are made to measure, or monitor, the flow of current within the ac power circuit. They're very useful in high power circuits, where the flow of current is large, i.e more current than the ratings of so-called self-contained current meters. Other applications relate to overcurrent and undercurrent relaying for power circuit protection, such as, within the power lead of an inverter or converter.
2.what do you mean by current transformer?
A current transformer is an electrical machine, that works to measure high AC which is not supposed to be sensed or passed through normal ammeters and current coils of wattmeter, energy meters, etc.
A current transformer basically includes a primary coil of single or more turns of heavy cross-sectional area. In some, the bar carrying a high current can act as a primary. This is joined in series with the circuit carrying a high current. The secondary of the AC transformer is constructed up from a large number of turns of fine wire having a small cross-sectional area. This is usually rated for Five ampere. This is coupled with the coil of the normal range ammeter.
4. What are the different types of current Transformers?
There are 5 types of current Transformers
1.Portable type
2.Outdoor type
3.Indoor type
4.Bushing type
5.Multi-ratio type
Indoor type current transformers are of two types
2.Bar and window type
Many outdoor type current transformers are actually used for higher voltage circuits. In the same way, Indoor types of current transformers are employed for low voltage circuits.
In a wound core type, the primary coil consisting of several turns is wound over the core. The core is mostly made up of a Ni-Fe alloy or specially treated steel and is used in such a way that the flux flowing always in the direction of grains as produced by Rolling the sheet metal. This improves the magnetic properties and helps in decreasing the ratio and phase angle errors.
Current Transformers using hot rolled Steel are made up of stacks of ring stampings which are insulated by means of the end-collars and circumferential wrap of pressboard protect the secondary winding from mechanical damage and also act as an insulating medium.
In a bar-type current transformer, the primary coil consists of a bar of suitable size that forms an integral part of the transformer as shown in figure 5.6. In bar type, the primary ampere-turns are numerically the same as the primary current. However, at a low current rating, the accuracy decreases because the magnetization of law demands a larger fraction of the total ampere-turns.
In the case of wound core type construction, higher accuracy can be achieved because of the higher value of primary ampere-turns.
The bushing type transformer is similar to the bar type. The core and secondary are mounted around a single primary conductor.
The secondary coil is placed over a circular core which is installed in the high voltage busing of a circuit breaker or power transformer.
The primary coil is the conductor in the bushing. In Precision type portable current Transformers, the choice of ratios can be obtained by changing the links on the top plate.
In laboratory-type current Transformers, the variable-ratio can be obtained by using the following methods:
1. The variable-ratio can be obtained by using the series-parallel connection of the primary coil. It should be ensured that the various coil sections used must have the same characteristics.
2. It can be obtained by using tappings on the primary or the secondary coil. This method provides a higher degree of flexibility. but when applied to the primary side, much of the copper space is left unused except on the lowest range. when used on the secondary-coil side, working ampere-turns are changed.
![]() |
Fig 5.6 CURRENT TRANSFORMER WITH BAR TYPE PRIMARY |
3.Using a variable primary formed by threading any desired number of turns of cable through the core. For this purpose, a ring core and a toroidal Core secondary is generally used.
It is also called a clamp-type ammeter in which the instrument and split-core transformer are combined to form a single unit. They have a self-aligning Hing Limb of split-core which is operated by a spring-loaded trigger to spread the core into two halves and clip around the current-carrying conductor as shown in figure 5.7.
![]() | |
|
This arrangement avoids the necessity of breaking the circuit for the purpose of measurement. For increasing the range shunts are used and by varying the shunt-resistance of the milli-ammeter circuit, current ranges from 0 to 5 ampere to 0 to 600 ampere can be obtained.
Nice, a very informative
ReplyDeleteWell written blog. very useful information. keep posting.
ReplyDeletePower Transformers in India | Transformer Manufacturer in India
Thanks for sharing this article as it enlighten us about types of Transformers. As a Transformer Manufacturers in India, we understands about the products and their uses as per the need. So, Our Team are able to manufacture customized transformers as per the requirement of client that met to national and international standards.
ReplyDeleteNice post with informative and good quality content. Thank you for sharing this information with us. This is really helpful. Also check control and relay panel manufacturers in Bangalore
ReplyDelete